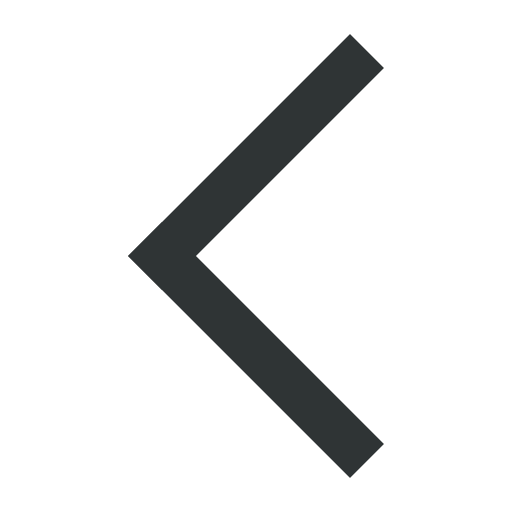
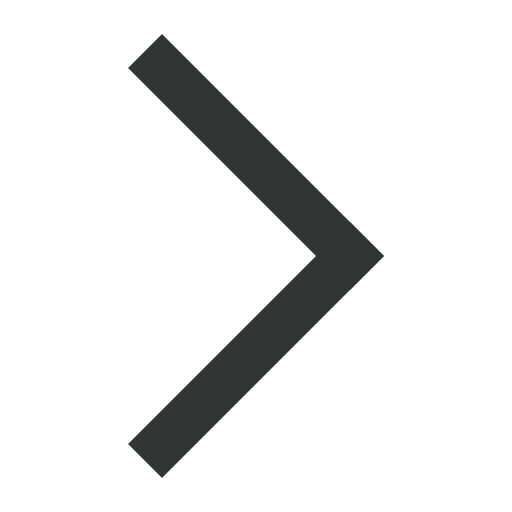

Research news
Lighting the way for Australian researchers: COVID-19 research at the Australian Synchrotron
![Fig1 [fig1]](https://www.iucr.org/__data/assets/image/0009/148527/fig1.jpg)
Figure 1. Ben Gully and Dene Littler at the Biomedicine Discovery Institute, Monash University.
As Australians returned to work from their summer holidays it became rapidly apparent that 2020 was going to be a year like no other. Faint reports of a strange new pneumonia in Wuhan, China, that had murmured in the global background while Australia endured the unprecedented summer bushfire season suddenly became a conflagration of their own. Australia identified its first case of confirmed COVID-19 on 25 January 2020, with events moving rapidly from that point onwards. Following Australian Government guidelines, the Australian Synchrotron moved to essential operations mode on Monday 23 March, cancelling all user experiments except for COVID-19-related research.
Like our sister synchrotrons around the world, we understood the vital importance of maintaining access to synchrotron radiation in driving structural-biology-related research into SARS-CoV-2 and made an early decision to reach out and support the efforts of our user community working in this field. In early March 2020 ANSTO/Australian Synchrotron offered a special rapid access mode to the MX2 Macromolecular Crystallography Beamline [1] for users undertaking COVID-19 research. This special rapid access mode would consist of short mini-shifts of beam time (a minimum of 2 hours), available on an “as-needed basis”. Proposal submission, review and user access processes would be fast-tracked. Our user community responded to the new special access mode with alacrity and agility, in many cases rapidly transitioning into a new field of research. We have been delighted with the varied and productive nature of the research.
The Australian Synchrotron’s user community ordinarily works across diverse fields of biology; this enabled many varied approaches to be undertaken with which to better understand SARS-CoV-2 and COVID-19 development, both in vitro and at a structural level, including research on both proteins from the SARS-CoV-2 virus alone and interactions between host and viral proteins.
The first SARS-CoV-2-related protein structure solved at the ANSTO/Australian Synchrotron was quickly published, and the resulting structure was made available immediately in the Protein Data Bank (PDB entry 6W9Q) by Dene Littler and colleagues from Monash University (Fig. 1) (Identification of a putative peptide-binding site at the dimer interface of the SARS-CoV-2 Nsp9 RNA-replicase. Littler, D. R., Gully, B. S. Colson, R. N. Riboldi-Tunnicliffe, A. & Rossjohn, J., to be published).
Dr Littler, working within the laboratory of Jamie Rossjohn, has focused on the parts of the virus produced inside human cells (the non-structural proteins; Nsps). These act to trick our body into becoming viral factories, and their enzymatic nature results in reduced genetic drift as new viral strains arise. Dr Littler is particularly interested in the molecular details of the less well understood Nsps, determining how they function in the context of infection and whether their action is amenable to small-molecule inhibition.
No approved drugs have yet been designed that specifically target coronaviruses but pharmaceutical companies around the world have begun assessing how pre-existing compounds might be productively repurposed for this role. The most promising targets to date are generally agreed as being the Nsp12 RNA-polymerase and the Nsp5 protease. As RNA viruses are prone to genetic drift, combination therapies can be required to target simultaneously different aspects of the virus. Dr Littler and his co-workers have obtained some interesting laboratory results for Nsp9, a small conserved viral protein that was thought to be involved in RNA binding, although it is unclear how it does so. They have identified a potential protein-binding site within Nsp9 and are working to better understand its significance (Fig. 2). The initial structure report has been published via bioRxiv [3].
![[Fig2]](https://www.iucr.org/__data/assets/image/0010/148528/fig2.jpg)
The laboratory of Joel MacKay has a proven track record in the research area of protein–protein interaction, and in particular the roles of these interactions in gene expression. A recent publication [4] revealed an important protein–protein interaction in cells infected with SARS-CoV-2.
To execute their program of replication, viruses hijack parts of the cellular machinery of their hosts. A prominent example of this phenomenon is the use that many viruses make of the host transcriptional apparatus to integrate and transcribe their genome. A recent analysis of the protein–protein interactome of the SARS-CoV-2 virus [4] has demonstrated that the viral envelope (E) protein can directly interact with the human proteins BRD2 and BRD4 (members of the BET family of bromodomain proteins). These bromodomains recognize acetylated lysines on histones and other proteins and are already promising therapeutic targets for the treatment of multiple conditions. These lead molecules available to date have moderate affinities and no paralogue specificity between BRD2, BRD4 and the related BRD3 and BRDT proteins.
In collaboration with Louise Walport from the Crick Institute in London and Hiroaki Suga in Tokyo, the MacKay laboratory is using an mRNA display of genetically reprogrammed cyclic peptides to design BET protein inhibitors with higher affinity and paralogue specificity. Their results to date demonstrate that cyclic peptides can be generated which display sub-nanomolar affinities with specificities that are much higher than has previously been observed [5]. They are currently exploring the structural and binding diversity of the highly diverse set of peptides that have been selected in their screens. X-ray crystallography has been a central tool in this exploration process, and Karishma Patel from the McKay Group (Fig. 3) has used the Australian Synchrotron to determine the structures of several of these peptides in complex with their bromodomain targets.
![[Fig3]](https://www.iucr.org/__data/assets/image/0011/148529/fig3.jpg)
The emergence of the SARS-CoV-2 virus in late 2019 provided a rare and unique opportunity for Stephanie Gras and her group at Monash University to expand their existing viral and structural immunology research interests and begin investigating the immune response towards an entirely novel virus. As a Viral and Structural Immunology group, Professor Stephanie Gras and her co-workers are focused on understanding T-cell responses towards different viruses through a structural lens. During a viral infection, viral peptides are presented from the immune system by proteins called human leukocyte antigens (HLA). These peptide–HLA (pHLA) complexes are displayed on the cell surface (like a flag) to be recognized by passing T cells. These T cells recognize the pHLA complex through their surface T-cell receptor (TCR), and the resulting immune response ensues. This process is complex, and Professor Gras knows from her previous work that understanding the presentation of peptides by HLA molecules and their interactions with T cells provides a wealth of knowledge about the resulting immune response. The ability to “see” the peptide presentation and recognition by the TCR using structural biology plays a significant role in understanding these interactions and is made possible utilizing X-ray crystallography at facilities such as the Australian Synchrotron. The expertise of the Gras group makes them ideally placed to understand the ability of the immune system to present various SARS-CoV-2 peptides (shared or not with SARS-CoV virus), and their ability to interact with the TCR. Understanding the ability and limitations of HLA molecules at presenting various SARS-CoV-2 derived peptides, their recognition by TCR and their impact on the immune response might provide valuable clues to aid in the design of effective therapeutics or a potential vaccine. Professor Gras’s group has solved the first structure of a SARS-CoV-2 peptide presented by an HLA molecule, and this will help them to better understand the T-cell response and participate in global efforts towards improving protective immunity against SARS-CoV-2.
The Commonwealth Science and Industry Research Organisation (CSIRO) is one of Australia’s premier research facilities and, as such, has been heavily involved in COVID-19 research since the beginning of the outbreak. One area of CSIRO’s research involving structural biology is being undertaken by Tom Peat and Alex Caputo. It is well established that viruses require host cellular functions in order to replicate and continue the infective process. As such, antiviral intervention can be targeted to either viral macromolecules (proteins or RNA/DNA) or specific host-cell proteins required for the viral life cycle. The latter strategy has advantages with respect to a larger barrier for drug resistance and the ability to target a broader range of viruses. Drs Peat and Caputo’s colleagues at CSIRO have determined a set of host cellular factors required for Hendra virus replication [6, 7]. This work has now been expanded to show that some of these host proteins are required for the replication of viruses beyond the paramyxoviridae family. Blocking one of these host proteins, human fibrillarin [8–11], an S-adenosyl-L-methionine (SAM) dependent nucleolar methyltransferase, has been shown to decrease the replication of a range of clinically important paramyxyoviruses, including the highly pathogenic Hendra and Nipah viruses by over 1000-fold, to the extent that virus replication is almost undetectable [10]. Drs Peat and Caputo performed a fragment screen by surface plasmon resonance (SPR) and crystallography to develop small-molecule inhibitors as antivirals. They discovered fragments with differing binding modalities and determined the crystal structures of several of these using the macromolecular crystallography beamlines at the Australian Synchrotron (Fig. 4). They are now in the process of building these fragments into drug-like compounds and determining whether these will be effective antiviral compounds for SARS-CoV-2.
![[Fig4]](https://www.iucr.org/__data/assets/image/0003/148530/fig4.png)
The work of Associate Professor Waihong Tham’s group at The Walter and Eliza Hall Institute (WEHI) aims to disrupt viral entry into host cells using nanobodies. Nanobodies are camelid-derived single-domain antibodies and represent the smallest naturally derived antigen-binding fragment (approximately 15 kDa in size). Their small size, along with high antigen-binding affinity, solubility, increased stability and ease of recombinant production (compared with traditional antibodies), make them attractive as potential therapeutics. These properties also allow the potential for the development of inhaled bio-therapies.
Entry of the SARS-CoV-2 virus is mediated by the interaction between the virus spike protein and its human receptor, angiotensin-converting enzyme 2 (ACE2). The high-affinity interaction with ACE2 is mediated by the receptor-binding domain (RBD) of the spike protein. To generate nanobodies, alpacas are immunized with virus spike and RBD proteins: the nanobody repertoire from isolated plasma cells is cloned into high-diversity phage display libraries, which are then used to select for antigen-specific nanobodies. The WEHI team will screen for nanobodies that inhibit the interaction of virus spike proteins with human ACE2, hence stopping virus entry and preventing SARS-CoV-2 infection.
Philip Pym and Melanie Dietrich are using the MX2 beamline of the Australian Synchrotron to determine the structure of the lead inhibitory nanobodies in complex with the RBD of SARS-CoV-2 to define key immune epitopes and to understand the structural mechanisms of inhibition. These structures will provide invaluable information that will allow further development of antibody therapies against SARS-CoV-2.
Conclusion
The ANSTO/Australian Synchrotron is committed to delivering research efforts into the SARS-CoV-2 virus, with our teams helping to address the impacts of COVID-19 via our national and landmark research platforms. One of the beamlines actively involved in SARS-CoV-2 and COVID-19 research, the Microfocus Crystallography beamline (MX2), has been used by several research teams to determine the structures of several proteins from the SARS-CoV-2 virus and/or related to our body’s response to COVID-19. The members of the scientific team employed by the ANSTO/Australian Synchrotron are highly experienced scientists with a diverse set of interests (from hands-on crystallography through to improving experimental techniques/outcomes using beamline equipment modification/improvement). The team consists of eight scientists who have rapidly responded to the immediate needs of our scientific community, as well as understanding the importance positive media has to play, informing the general public of the work being undertaken both within Australia and the world in general. All team members are proud to be playing our vital part in one of the greatest public health and societal challenges of our time.Access requests for researchers undertaking SARS-CoV-2/COVID-19 can be submitted via the special COVID-19 access round (https://portal.synchrotron.org.au), selecting MX1: Macromolecular Crystallography [12] as the equipment set ID and then Special Rapid Access COVID-19 (for the round).
For commercial (paid) access to undertake research at the Australian Synchrotron please contact Dr David Cookson (cooksond@ansto.gov.au).
References
[1] Aragão, D., Aishima, J., Cherukuvada, H., Clarken, R., Clift, M., Cowieson, N. P., Ericsson, D. J., Gee, C. L., Macedo, S., Mudie, N., Panjikar, S., Price, J. R., Riboldi-Tunnicliffe, A., Rostan, R., Williamson, R. & Caradoc-Davies, T. T. (2018). MX2: a high-flux undulator microfocus beamline serving both the chemical and macromolecular crystallography communities at the Australian Synchrotron. J. Synchrotron Rad. 25, 885–891.
[2] Littler, D. R., Gully, B. S., Colson, R. N. & Rossjohn, J. (2020). Crystal structure of the SARS-CoV-2 non-structural protein 9, Nsp9, ISCIENCE, https://doi.org/10.1016/j.isci.2020.101258.
[5] Patel et al. (2020). BioRxiv, https://www.biorxiv.org/content/10.1101/850321v1.
Rachel M. Williamson and Alan Riboldi-Tunnicliffe are Principal Scientists at the Australian Synchrotron (ANSTO), Clayton, VIC, Australia.
Copyright © - All Rights Reserved - International Union of Crystallography