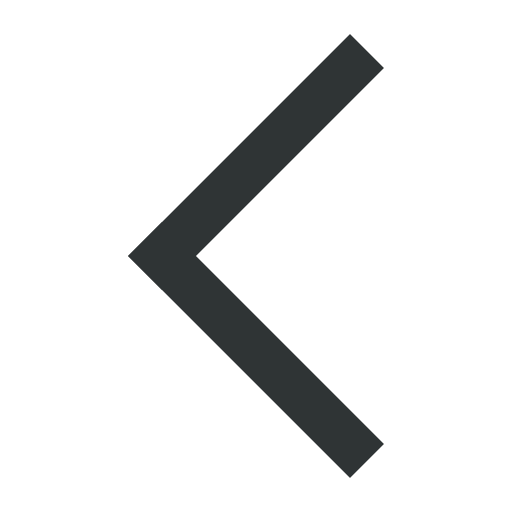
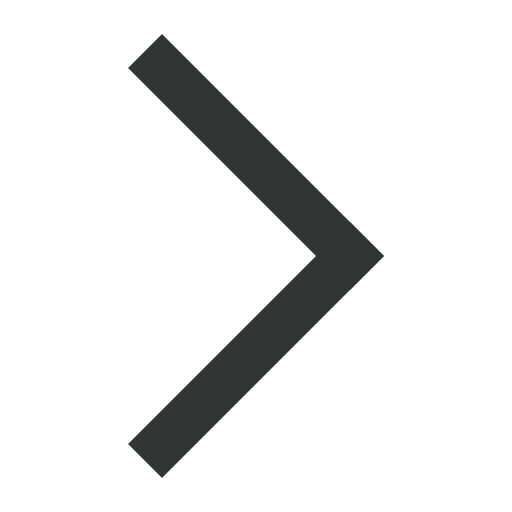

Research news
Supporting COVID-19 research at the NSLS-II
![Figure1 [Figure1]](https://www.iucr.org/__data/assets/image/0006/148605/Figure1.png)
Figure 1. Endstation of one of the MX beamlines (AMX, 17-ID-1).
The National Synchrotron Light Source II (NSLS-II) at Brookhaven National Laboratory (BNL), Upton, NY, is the latest and brightest synchrotron facility in operation in the US. As of May 2020, it remains in a special user-operation mode to support exclusively COVID-19 research. All DOE BES Synchrotron facilities have re-scheduled their planned maintenance shutdowns to provide continuous access to X-ray beams for life sciences experiments in the US. As a result, the NSLS-II will remain in extended operation until mid-June 2020. The NSLS-II supports research from 29 operating beamlines and, under the current special circumstances, maintains normal operation for three dedicated beamlines.
Two macromolecular crystallography (MX) beamlines and one dedicated life-science X-ray scattering beamline accept Rapid Access proposals for COVID-19 related experiments. To maintain continuous safe operation, all experiments are performed remotely with minimum staff at the beamline to provide support.
The highly Automated and Frontier MX beamlines (AMX and FMX, respectively) (Fuchs et al., 2016) offer remote access and automated data collection, providing state-of-the-art capabilities in terms of X-ray beam (size and flux) and rapid screening for potential crystals as small as 1 μm (Fig. 1). Most of the efforts at the MX beamlines are either to support or implement research intended to determine the structures of proteins from the Severe Acute Respiratory Syndrome Coronavirus 2 (SARS-CoV-2). The major targets have been the spike protein and the main protease, which happen to be studied by most groups worldwide.
Operating the three beamlines safely and at full performance, with minimal staff observing social distancing, and without the possibility for users to come on site requires several changes. All users conduct their experiments remotely through a NoMachine session. Users that access the beamlines for the first time have dedicated training sessions one week before their actual beam time, to ensure their connection performance and help them make full use of the beamlines’ capabilities and beam specifications. During a user experiment, only one staff member is present at the beamline, with engineering and technical support on call if needed. In daily walkthroughs and regular test experiments, we confirm that all systems are running optimally and establish the beamline performance. Newly developed monitoring systems and remotely accessible websites developed by the accelerator division of the NSLS-II greatly facilitate supporting and observing systems from outside the laboratory.
In order to further accelerate early discoveries of potential diffracting conditions from either microcrystals or poorly diffracting samples that are typically produced in the very early stages of drug-discovery projects, we have adapted one of our existing data collection protocols to deliver improved results to highlight possible hits. In short, the pipeline evaluates the fast raster data in an initial spot-finder run and attempts to index frames with a number of reflections above a threshold. Successfully indexed frames with the smallest cell dimensions above a threshold are highlighted. Planned updates include further tuning and graphical presentation of the scan results.
NSLS-II started minimum safe operation mode, where only the two MX beamlines were in operation in March of 2020 and soon authorized the SAXS beamline to also enter operation under the same conditions (COVID-19-related work). So far we have supported 20 experimental visits from several proprietary groups and started in-house research as well.
In-house/collaboration research
Spike protein: membrane fusion
Structure-guided development of viral fusion inhibitors: after the S protein receptor-binding domain (RBD) has bound to the angiotensin-converting enzyme 2 (ACE-2) receptor, the heptad repeat 1 (HR-1) and 2 (HR-2) domains interact with each other to form a six-helix bundle (Tang et al., 2020: 6HB; Fig. 2). This conformational rearrangement brings the viral envelope into proximity with the host-cell membrane and facilitates membrane fusion. This type I fusion mechanism, employed by other viruses such as HIV, Ebola and RSV, presents a viable target for the treatment or prevention of infection by SARS-CoV-2 (Tang et al., 2020). Strategies that aim to exploit this type of fusion mechanism mainly involve the development of synthetic variants of the S protein HR-2 domain (or equivalent), which bind to the HR-1 domain (or equivalent) trimer and disrupt the formation of the 6HB (Tang et al., 2020). These fusion inhibitory HR-2 domain variants are typically large polypeptides in excess of 3 kD which form an extensive interface with a protein surface; therefore, structural information plays an integral role in expediting the development process.
![[Figure2]](https://www.iucr.org/__data/assets/image/0007/148606/Figure2.png)
Recently, Lu et al. published the structure of the SARS-CoV-2 6HB (PDB entry 6LXT) in addition to describing a fusion inhibitor peptide with broad-spectrum activity against multiple human coronaviruses, laying the groundwork for the development of SARS-CoV-2 fusion inhibitors derived from the S protein HR-2 domain (Lu et al., 2020) (Fig. 3). Currently, work is under way at NSLS-II to provide structural support in a collaborative effort with molecular dynamics (MD) and chemists at academic institutions to generate peptide fusion inhibitors with improved inhibitory activity and/or pharmacokinetic properties. The in-house crystallization laboratory, adjacent to the MX beamlines, has allowed for the immediate screening of potential lead conditions, and the microbeams and raster processing pipelines have been essential for the rapid identification and indexing of microcrystals from initial sparse matrix hits.
![[Figure3]](https://www.iucr.org/__data/assets/image/0008/148607/Figure3.png)
Spike protein receptor-binding domain
The SARS-CoV-2 spike protein induces cellular infection via a multi-step process, with different steps carried out by different domains. The spike protein is the only extracellular COVID-19 therapeutic target. The spike protein RBD is responsible for initial recognition between the spike protein and the human ACE-2. This makes RBD an attractive target for antiviral drug development. The RBD domain has been biochemically characterized, and crystal structures are available for RBD bound to monoclonal antibodies (PDB entries 6YLA, 6W41) and bound to ACE-2 (PDB entries 6LZG, 6M17). Low-resolution cryo-EM structures are available for the full-length spike protein trimer in the closed conformation (PDB entry 6VXX), in the open conformation (PDB entry 6VYB) and in a mixed state (PDB entry 6VSB). At the time of writing, there is no crystallographic structure known of the RBD trimer.
To effectively use fragment screening as a search tool for potential COVID-19 antivirals, we are searching for crystallization conditions for RBD unbound to antibodies or ACE-2. We are attempting crystallization trials for three RBD constructs. We have begun crystallization trials for the full-length 239 amino acid RBD domain. Additional crystallization trials are ongoing for a reduced-length 193aa construct with an N-terminal truncation, and for a 131aa construct with both N- and C-terminal truncations (the shortened constructs were designed to improve crystallization characteristics such as stability and solubility). Once reliable crystallization conditions are established, we will use structure-based fragment screening as a technique to identify potential antivirals which target SARS-CoV-2 RBD.
Main protease
The SARS-CoV-2 genome consists of ~30,000 nucleotides, and its replicase gene encodes two overlapping polyproteins, pp1a and pp1ab, which are required for viral replication and transcription (Zhou et al., 2020; Wu et al., 2020). These two polyproteins undergo extensive proteolytic processing largely by the main protease (Mpro), also referred to as the 3-chymotrypsin-like cysteine protease (3CLpro). Mpro has a Cys–His catalytic dyad at the active site and cleaves 11 sites in the p1 position of pp1a and pp1ab (Shimamoto et al., 2015). This cleavage process produces a mature protein that anchors the replication/transcription complex and releases the mature non-structural proteins (NSPs) (Hsu et al., 2005; Barretto et al., 2005). Inhibition of Mpro prevents the production of mature proteins required for further replication, which makes it an attractive drug target.
The structure of Mpro with its mechanism-based inhibitor has been reported (Jin et al., 2020), and this study gave us a starting point to develop more potent inhibitors against the Mpro activity. Using a structure-based drug design (SBDD) approach, we screened the FDA-approved drug database and selected potential antiviral and pathogen-specific hits. We are screening the selected drugs for inhibition, and the positive candidates will be subjected to co-crystal structure determination. In addition, we have determined the higher-resolution crystal structure of Mpro in a native conformation (Fig. 4). In the crystal structure, the active site of Mpro is open to the solvent region, and it is in a favorable form for the soaking and co-crystallization experiments. Our goal is to derive the structure–activity relationship (SAR) to identify drug modifications that improve potency and efficacy through multiple rounds of design, synthesis and in vitro/in vivo testing.
![[Figure4]](https://www.iucr.org/__data/assets/image/0009/148608/Figure4.png)
Research carried out by pharmaceutical users
NSLS-II has hosted several companies that are researching treatments for COVID-19, with more companies planning to use the beamlines as early as possible. These companies are requesting repeated access. New protocols have been established to dedicate all resources to COVID-19-related research, to fast track approval of new COVID-19 projects and to ensure that data can be safely obtained from COVID-19-related samples. The approved projects tested a wide variety of strategies such as antiviral drugs that interfere with the viral infection and drugs that boost the immune system before infection and heal injuries post-infection. To date, more than 1000 samples have been screened, 40% of them have provided useful data (processed files).
The metadata from proprietary users indicate a significant change in the usage pattern during the COVID-19 crisis. For example, companies are bringing more samples related to the early stages of research, and fewer samples from mature projects (preliminary research has a high proportion of samples rejected during diffraction screening). Proprietary users are applying for beam time to test microcrystals in the size range 2–5 μm from difficult-to-crystalize targets or from initial crystallization screenings. This change in usage pattern confirms that our new protocols for expedited approval are encouraging proprietary users to take advantage of the unique micro-focusing and automation capabilities of NSLS-II at the earliest possible stage in their drug development work flow.
Research carried out at the bioSAXS beamline
There is a demand for solution scattering at the LiX beamline to support COVID-19 research as well. Solution scattering provides low-resolution structural information when the molecule or molecular complex cannot be crystallized or needs to be studied under near-physiological conditions. So far, the LiX beamline has scheduled two user experiments through COVID-19 Rapid Access proposals. More experiments, including those in support of the in-house research described above, are under discussion. In addition to the capabilities for high-throughput measurements and in-line size-exclusion chromatography, the ability to collect data up to wide scattering angles (q ~ 3.2 Å-1) (Yang et al., 2020) is of particular interest to these users, to detect molecular binding (Fischetti et al., 2004).
Research carried out using computer modeling on the NSLS-II/CBMS cluster
Additionally, CBMS and NSLS-II computing resources, when not in use for ongoing data collection at the two MX beamlines, are made available using the SLURM Workload Manager system to other groups from NSLS-II. These roughly 700 cores are currently part of a large collaborative project to target the entire proteome of SARS-CoV-2 to identify small molecules with positive binding.
Using physics-based and artificial intelligence (AI)-driven virtual screening models, and AI-enhanced MD to further assess binding, the most promising molecule and SARS-CoV-2 protein complexes will be investigated experimentally, including structural biology studies. Nodes are used every night, every weekend and every day that the beamline is not operating.
We particularly thank the NSLS-II accelerator division staff members who maintain the continuous operation of the storage ring to support user and staff research and develop new monitoring systems and control systems for remote operation. We also thank the ICT group from NSLS-II who continuously maintain stable operation of the file system and our small dedicated cluster.
We appreciate that in times like these, operating the light source safely and supporting our work is a difficult undertaking, and we are very thankful for the continued support by the technical, engineering and operations groups.
We thank the Diamond Light Source for sending the plasmid for the main protease and for sharing protocols for expression, purification and crystallization.
Work on the Mpro is a collaboration with John Shanklin from the Biology Department at BNL, NY. The computing work is led by Hubertus Van Dam at the Computing Science Initiative, BNL, NY.
The work on the spike protein membrane fusion is a collaboration with Victor Outlaw and Sam Gellman from the University of Wisconsin-Madison and with Matteo Porotto and Anne Moscona from Columbia University.
References
Barretto et al. (2005). J. Virol. 79, 15189–15198. https://doi.org/10.1128/JVI.79.24.15189-15198.2005
Fischetti et al. (2004). Cell Chem. Biol. 11, 1431–1433. http://doi.org/10.1016/j.chembiol.2004.08.013
Fuchs et al. (2016). AIP Conf. Proc. 1741, 030006. http://doi.org/10.1063/1.4952829
Hsu et al. (2005). J. Biol. 280, 31257–31266. http://doi.org/10.1074/jbc.M502577200
Jin et al. (2020). Nature, 582, 289–293. https://doi.org/10.1038/s41586-020-2223-y
Lu et al. (2020). Cell Res. 30, 343–355. http://doi.org/10.1038/s41422-020-0305-x<</a>
Shimamoto et al. (2015). Bioorg. Med. Chem. 23, 876–890. https://doi.org/10.1016/j.bmc.2014.12.028
Tang et al. (2020). Antivir. Res. 178, 104792. https://doi.org/10.1016/j.antiviral.2020.104792
Wu et al. (2020). Nature, 579, 265–269. https://doi.org/10.1038/s41586-020-2008-3
Yang et al. (2020). J. Synchrotron Rad. 27, 804–812. https://doi.org/10.1107/S1600577520002362
Zhou et al. (2020). Nature, 579, 270–273. https://doi.org/10.1038/s41586-020-2012-7
The authors are at the National Synchrotron Light Source II, Brookhaven National Laboratory, Upton, NY, USA, and the Biology Department, Brookhaven National Laboratory, Upton, NY, USA.
Copyright © - All Rights Reserved - International Union of Crystallography