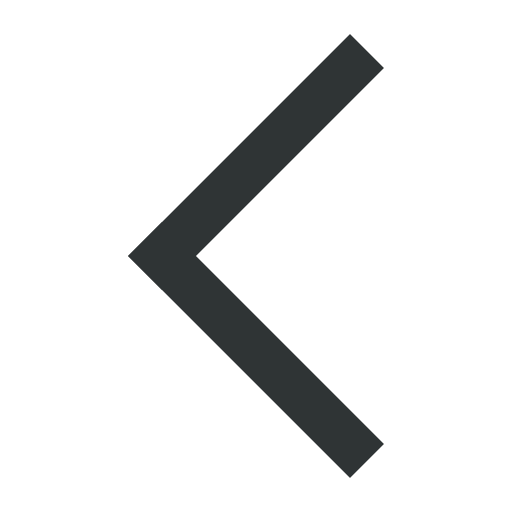
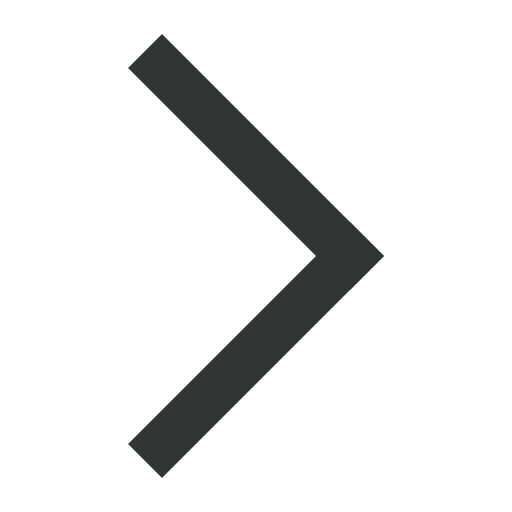

IUCr journals news
Introduction to the virtual thematic issue on room-temperature biological crystallography
![Thumbnail [Thumbnail]](https://www.iucr.org/__data/assets/image/0008/156869/me6219thumbnail.png)
After many years in which the use of cryogenic conditions (typically around 100 K) represented an almost ubiquitous approach to biological crystallographic studies, there has been a recent resurgence in room temperature (RT) or (near-)physiological temperature experimentation. One of the main advantages of measuring diffraction data at low temperatures is the possibility of mitigating the detrimental effects of radiation-induced damage in macromolecular crystals (Garman & Schneider, 1997). Although cryo-conditions do not completely abolish global and specific radiation damage (Bui et al., 2014; Garman, 2010; Ravelli & McSweeney, 2000; Weik et al., 2000), their use, together with judicious instrument choices, often allow the collection of complete diffraction data from a single crystal without significant loss in resolution. Nowadays, whilst the ‘one crystal, one data set’ experimental format afforded by cryo-temperatures remains popular and practically convenient, advances in multi-crystal serial data collection approaches (SFX and SSX for serial femtosecond and serial synchrotron X-ray crystallography, respectively) and detectors with extremely fast read-out rates allow the measurement of complete datasets at RT with modest radiation-induced damage. These developments offer exciting opportunities as there is an increasing realization that RT or multi-temperature measurements, represent a valid approach to study functionally important conformations that may be hidden at cryogenic temperatures (Fraser et al., 2009; Keedy et al., 2018; Keedy et al., 2015; Keedy et al., 2014; Fenwick et al., 2014). Also, temperature-dependent differences in protein–ligand interactions including unique binding poses and new binding sites (Skaist Mehlman et al., 2023) make RT crystallographic studies very relevant for a more complete understanding of protein–ligand interactions that might be important for drug discovery (Fischer et al., 2014; Fischer et al., 2015). Importantly, RT studies on microcrystals coupled with suitable mixing and/or activation methods can also allow the visualization of reaction intermediates (Olmos et al., 2018; Butryn et al., 2021; Gruhl et al., 2023).
This virtual thematic issue (see https://journals.iucr.org/special_issues/2022/RT/) presents a collection of articles recently published in IUCrJ, Acta Cryst. D Structural Biology and Acta Cryst. F Structural Biology Communications, some of which have been commissioned specifically, that highlight topics and trends in modern RT biological crystallography.
In a review paper, Thorne provides an overview of the key advantages and physical principles of, and methods for, crystallographic data collection at non-cryogenic temperatures (Thorne, 2023). The paper also discusses some factors relevant to interpreting the resulting data. This review complements another excellent review on practical methods for RT data collection and analysis published recently in Quarterly Reviews of Biophysics (Fischer, 2021).
A series of original research articles then discuss RT crystallographic work in the context of different biological systems. Metal centres in proteins can be very sensitive to radiation damage thus making standard single-crystal RT experiments particularly challenging. Moreno-Chicano and co-workers explored the complementarity of several diffraction techniques to study the multifunctional heme-containing dehaloperoxidase B (DHP-B) globin (Moreno-Chicano et al., 2022). Aumonier and colleagues used RT time-resolved oscillation crystallography coupled with in crystallo UV–Vis absorption spectroscopy to look at the slow relaxation of a reaction intermediate – a thio-ether covalent bond between the chromophore and a cysteine residue – in the photoreaction of the LOV2 domain of phototropin 2 from Arabidopsis thaliana (Aumonier et al., 2022). Ebrahim and co-workers performed a multi-temperature experiment on the SARS-CoV-2 main protease (Mpro), a promising target for the development of novel antiviral therapeutics (Ebrahim et al., 2022). Using multi-copy ensemble models they highlight conformational heterogeneity at various sites including a key substrate-binding loop that may inspire new strategies for antiviral drug development. Sharma and co-workers further explored the theme of conformational variability and allosteric coupling in protein tyrosine phosphatase 1B (PTP1B) by solving its SSX structure in the unliganded state (Sharma et al., 2023) whilst Schneps and colleagues employed SSX on the circadian photoreceptor cryptochrome (CRY) revealing regions of enhanced mobility in loops that have functional importance within this protein family and whose atomic displacement parameters correlate well with those predicted from molecular-dynamics simulations (Schneps et al., 2022). The theme of ligand-binding discrepancies between cryo-cooled and physiological temperature structures is discussed by Huang and co-workers by looking at the interaction of TL00150, a 175.15 Da fragment, with endothiapepsin using a ‘temperature-resolved’ approach (Huang et al., 2022). Some aspects of radiation damage are considered by Yabukarski and colleagues who employed a panel of well known model systems, to show that radiation damage only modestly increases conformational heterogeneity at RT, suggesting that dynamic information can be obtained from single crystals at near-physiological temperature (Yabukarski et al., 2022). Båth and co-authors employed lipidic cubic phase (LCP) microcrystallization applied to the photosynthetic reaction centre of Blastochloris viridis (Båth et al., 2022). Using X-ray free electron laser (XFEL) radiation they obtained its structure at the 2.25 Å resolution that allowed the visualization of the mobile ubiquinone potentially facilitating time-resolved diffraction studies of electron-transfer reactions to this cofactor. Finally, Zielinski and co-workers discussed efficient RT SSX experiments carried out on three different proteins of biological relevance using the CFEL TapeDrive, a conveyor-belt-based sample-delivery system (Zielinski et al., 2022).
The issue also includes a few articles that are a bit more ‘technical’ in their nature. Greisman and colleagues discuss native single anomalous diffraction (SAD) phasing at RT (Greisman et al., 2022) whilst Doukov and co-workers show how high-quality anomalous signal can be collected from single-crystal diffraction data with relatively low occupancy at and above RT (Doukov et al., 2023). In the field of synchrotron instrumentation, increasingly more sophisticated developments are happening at sites worldwide. One example is discussed by Okumura and colleagues in their presentation of the setup for in situ crystal data-collection and ligand-screening available at SPring-8 (Okumura et al., 2022).
Hopefully, this virtual thematic issue will encourage the reader to explore additional published work on the topic of RT biological crystallography and, importantly, facilitate the conception and execution of relevant experiments.
References
Bui, S., von Stetten, D., Jambrina, P. G., Prangé, T., Colloc’h, N., de Sanctis, D., Royant, A., Rosta, E. & Steiner, R. A. (2014). Angew. Chem. Int. Ed. 53, 13710–13714.
Butryn, A., Simon, P. S., Aller, P., Hinchliffe, P., Massad, R. N., Leen, G., Tooke, C. L., Bogacz, I., Kim, I. S., Bhowmick, A., Brewster, A. S., Devenish, N. E., Brem, J., Kamps, J., Lang, P. A., Rabe, P., Axford, D., Beale, J. H., Davy, B., Ebrahim, A., Orlans, J., Storm, S. L. S., Zhou, T., Owada, S., Tanaka, R., Tono, K., Evans, G., Owen, R. L., Houle, F. A., Sauter, N. K., Schofield, C. J., Spencer, J., Yachandra, V. K., Yano, J., Kern, J. F. & Orville, A. M. (2021). Nat. Commun. 12, 4461.
Doukov, T., Herschlag, D. & Yabukarski, F. (2023). Acta Cryst. D79, 212–223.
Fenwick, R. B., van den Bedem, H., Fraser, J. S. & Wright, P. E. (2014). Proc. Natl Acad. Sci. USA, 111, E445–E454.
Fischer, M. (2021). Q. Rev. Biophys. 54, e1.
Fischer, M., Coleman, R. G., Fraser, J. S. & Shoichet, B. K. (2014). Nat. Chem. 6, 575–583.
Fischer, M., Shoichet, B. K. & Fraser, J. S. (2015). ChemBioChem, 16, 1560–1564.
Fraser, J. S., Clarkson, M. W., Degnan, S. C., Erion, R., Kern, D. & Alber, T. (2009). Nature, 462, 669–673.
Garman, E. F. (2010). Acta Cryst. D66, 339–351.
Garman, E. F. & Schneider, T. R. (1997). J. Appl. Cryst. 30, 211–237.
Gruhl, T., Weinert, T. & Rodrigues, M. J. (2023). Nature, https:// doi.org/10.1038/s41586-023-05863-6.
Keedy, D. A., Hill, Z. B., Biel, J. T., Kang, E., Rettenmaier, T. J., Brandao-Neto, J., Pearce, N. M., von Delft, F., Wells, J. A. & Fraser, J. S. (2018). eLife 7, e36307.
Keedy, D. A., Kenner, L. R., Warkentin, M., Woldeyes, R. A., Hopkins, J. B., Thompson, M. C., Brewster, A. S., Van Benschoten, A. H., Baxter, E. L., Uervirojnangkoorn, M., McPhillips, S. E., Song, J., Alonso-Mori, R., Holton, J. M., Weis, W. I., Brunger, A. T., Soltis, S. M., Lemke, H., Gonzalez, A., Sauter, N. K., Cohen, A. E., van den Bedem, H., Thorne, R. E. & Fraser, J. S. (2015). eLife 4, e07574.
Keedy, D. A., van den Bedem, H., Sivak, D. A., Petsko, G. A., Ringe, D., Wilson, M. A. & Fraser, J. S. (2014). Structure, 22, 899–910.
Olmos, J. L. Jr, Pandey, S., Martin-Garcia, J. M., Calvey, G., Katz, A., Knoska, J., Kupitz, C., Hunter, M. S., Liang, M., Oberthuer, D., Yefanov, O., Wiedorn, M., Heyman, M., Holl, M., Pande, K., Barty, A., Miller, M. D., Stern, S., Roy-Chowdhury, S., Coe, J., Nagaratnam, N., Zook, J., Verburgt, J., Norwood, T., Poudyal, I., Xu, D., Koglin, J., Seaberg, M. H., Zhao, Y., Bajt, S., Grant, T., Mariani, V., Nelson, G., Subramanian, G., Bae, E., Fromme, R., Fung, R., Schwander, P., Frank, M., White, T. A., Weierstall, U., Zatsepin, N., Spence, J., Fromme, P., Chapman, H. N., Pollack, L., Tremblay, L., Ourmazd, A., Phillips, G. N. Jr & Schmidt, M. (2018). BMC Biol. 16, 59.
Ravelli, R. B. & McSweeney, S. M. (2000). Structure, 8, 315–328.
Schneps, C. M., Ganguly, A. & Crane, B. R. (2022). Acta Cryst. D78, 975–985.
Sharma, S., Ebrahim, A. & Keedy, D. A. (2023). Acta Cryst. F79, 23–30.
Skaist Mehlman, T., Biel, J. T., Azeem, S. M., Nelson, E. R., Hossain, S., Dunnett, L., Paterson, N. G., Douangamath, A., Talon, R., Axford, D., Orins, H., von Delft, F. & Keedy, D. A. (2023). eLife 12, e84632.
Thorne, R. E. (2023). Acta Cryst. D79, 78–94.
Weik, M., Ravelli, R. B., Kryger, G., McSweeney, S., Raves, M. L., Harel, M., Gros, P., Silman, I., Kroon, J. & Sussman, J. L. (2000). Proc. Natl Acad. Sci. USA, 97, 623–628.
This article was originally published in Acta Cryst. (2023). D79, 268–270; Acta Cryst. (2023). F79, 79–81; and IUCrJ (2023). 10, 248–250.
Copyright © - All Rights Reserved - International Union of Crystallography