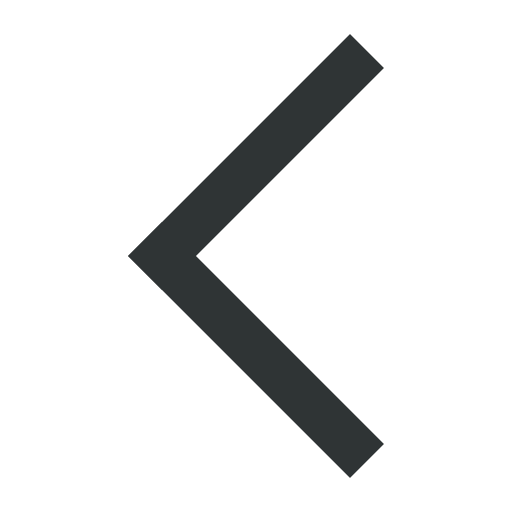
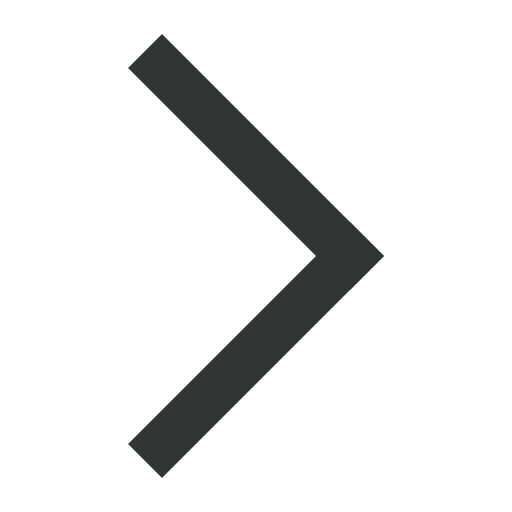

Feature article
Pauling's rules revisited
![Thumbnail [Thumbnail]](https://www.iucr.org/__data/assets/image/0019/156610/Thumbnail.jpg)
Almost a century ago, Linus Pauling (1929) proposed a set of rules governing the structural chemistry of inorganic crystals. The rules were a tentative step toward creating a valence theory for inorganic compounds. Unfortunately, the new quantum mechanics that promised to answer all of chemistry’s questions appeared at the same time, and Pauling’s rules were soon forgotten. They are worth a second look because they point to a simple but rich theory of valence that describes the chemistry of both organic and inorganic compounds using only the simple concepts of valence, atoms and bonds.
Curiously, for all its success, quantum mechanics has never provided definitions for either bonds or valence, or even for atoms once they are incorporated into a molecule. The reason for this failure is simple. Quantum mechanics calculates the energy and other physical properties using the electric field that connects electrons to nuclei, but any calculation using a force field is a mathematical integration that requires a set of initial conditions, such as the spatial coordinates of the nuclei. This restricts each calculation to a single spatial arrangement of atoms. Valence theories are more flexible because they do not use atomic coordinates. Instead, they define the location of an atom by its place within the network of bonds that links the atom to its neighbours. Chemical properties such as molecular structure and reactivity are determined by how the network distributes the valences of the atoms among its various bonds. By not having to specify atomic coordinates, valence theories are simpler and more intuitive, but at the cost of being unable to calculate energies. It follows that quantum and valence approaches provide complementary descriptions of the same compound. Quantum theory describes the physics of electrons bound to nuclei, valence theory describes the chemistry of atoms linked by bonds. In the past, when the atom was still just a hypothesis, valence theory brought order to organic chemistry where it is still widely used, but finding a comparable theory of inorganic chemistry has proved elusive.
Pauling presented his rules in an early attempt to formulate an inorganic valence theory. His first two rules are visionary because they express the idea that structural chemistry is largely determined by the sizes and valences of the atoms, concepts that serve as surrogates for the quantum picture of the core and the valence shell, respectively. Hidden unnoticed within these rules lie the clues to a unified theory of valence, one that provides an enriched description of the structure and properties of both organic and inorganic compounds.
Pauling’s first rule states that the coordination number of a cation depends on the ratio of the cation to anion radii, but all attempts to define the radius of an atom or ion have failed because atoms do not behave like hard spheres. What has hitherto been overlooked is that the proper measure of atomic size in chemistry is not the radius of the atom, which is undefinable, but the coordination number that the atom adopts in different chemical contexts. The elemental property that best characterizes the size of an atom is the average of its observed coordination numbers.
![[Fig. 1]](https://www.iucr.org/__data/assets/image/0020/156611/Picture1.jpg)
Pauling’s second rule defines the bond strength of a cation as its valence divided by its coordination number. The rule states that the valence of an anion is approximately equal to the sum of the bond strengths it receives from its cation neighbours. This rule introduces four important ideas:
- Even though the ionic model uses an electric force field to link cations to anions, Pauling assumes that this field can be transformed into a network of localized bonds. A formal derivation shows that while such a transformation is possible, it is not reversible because the atomic coordinates are lost in the process.
- The most important chemical property of an atom is neither its valence nor its coordination number, but its bond strength which indicates its preferred bond partners.
- Two atoms contribute equal valences to form a bond.
- The bond strength, being a ratio, is not restricted to integral values. The traditional association of valence with an electron has no physical basis. Valence and electrons can never be measured at the same time: the valence of an atom can only be measured when it is part of a compound, but an individual electron can only be observed when it is an isolated particle.
A unified theory of valence is possible if non-integral valences are allowed. It describes the chemical structures of both organic and inorganic compounds by a network of localized bonds that obey a number of simple rules. Elements are characterized by their observed properties of valences and average coordination number, but their most important property is their bonding strength, equal to the ratio of their valence to average coordination number. Bonds are found to form only between atoms whose bonding strengths differ by less than a factor of two, though atoms with lone pairs, such as anions, can form stronger bonds by making their lone pairs stereoactive, thus accounting for the different chemistries of anions and cations. The ionicity of a bond is the difference between the electronegativities of its bonded atoms, where the electronegativity can be shown to be equal to the bonding strength of the element in its highest valence state. Organic molecules are formed by atoms with bonding strengths close to 1.0, allowing them to form bonds of low ionicity in many different ways. Hydrogen and fluorine necessarily terminate the bond network to form discrete molecules.
The resulting unified valence theory (Brown, 2023) is quantitative and can predict which atoms will form bonds, as well as the bond lengths, geometries and chemical properties of the resulting compound. The structure and chemistry of hydrogen bonds, and likely also of halogen bonds, are predicted from atomic valences and sizes. The theory describes solubilities and the species present in aqueous solutions at various pHs. Where practical considerations often limit quantum mechanical calculations to isolated clusters of atoms, valence theory incorporates a knowledge of the chemical environment and its influence on the internal structure of a molecule. Even though valence theory cannot determine physical properties, its simplicity makes it an ideal way to introduce students to chemistry, and for those who later graduate to quantum mechanics, it provides a way of selecting a structure for a more comprehensive quantum investigation.
References
Pauling, L. (1929). The principles determining the structure of complex ionic crystals. J. Am. Chem. Soc. 51, 1010–1026.
Brown, I.D. (2023). A rigorous theory of valence. Struct. Chem. 34, 361–389.
I. David Brown is Professor Emeritus at Brockhouse Institute for Materials Research, McMaster University, Hamilton, ON, Canada; idbrown@mcmaster.ca.
Copyright © - All Rights Reserved - International Union of Crystallography