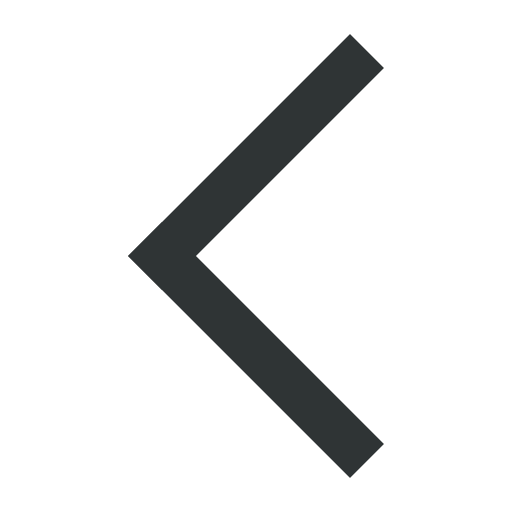
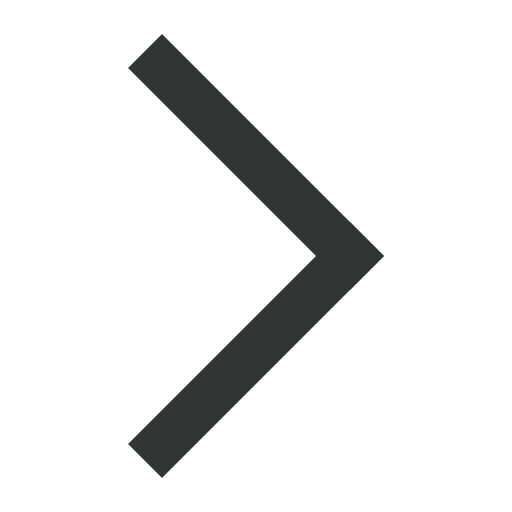

Feature article
MAX IV Laboratory – a fourth-generation light source
![Thumbnail [Thumbnail]](https://www.iucr.org/__data/assets/image/0016/155122/Thumbnail.jpg)
Early morning at MAX IV, located in Lund, Sweden. Neighbouring neutron facility European Spallation Source, ESS, is visible in the upper right corner.
MAX IV Laboratory is a Swedish national synchrotron laboratory that has been operating as a user facility since 2016. It is the successor to MAX-lab, which was in operation between 1987 and 2015. It offers access to 16 beamlines that provide modern X-ray spectroscopy, scattering/diffraction and imaging techniques to contribute to solving scientific questions. The latest additions to our beamline portfolio are ForMAX and MicroMAX.
MAX IV welcomes about 1000 users from academia and industry every year, which is expected to double in the coming years. About 75% of our users are affiliated with institutions from Nordic countries (Sweden, Denmark, Norway, Finland, Iceland). Our beamlines are open to academia, research institutes, industry and government agencies worldwide. Access is without charge for users who intend to publish their results in the open literature. It is granted based on the scientific merit and technical feasibility of science proposals submitted twice a year. Industry can also get access through a proprietary access program.
MAX IV is currently formulating a strategy for the upcoming years towards 2035 in collaboration with the user community. Our strategy will identify opportunities to address important scientific questions of high relevance to society that will translate into the definition of a set of new beamlines to continue to develop our beamline portfolio.
The recently opened ForMAX beamline focuses on renewable materials
One of the newest additions to MAX IV is the ForMAX beamline that recently opened for users. A large part of the research at ForMAX will focus on developing materials and chemicals from renewable supplies and forest-based sources. ForMAX is financed by industry, universities and foundation partners in the Treesearch consortium.
An important focus area of ForMAX is characterising complex materials across a broad range of length scales. For example, the mechanical properties of wood originate from various hierarchical structures and sub-structures spanning from hundreds of micrometres down to the nanometre scales. This will be performed by combining full-field tomography with small- and wide-angle scattering. The experiment setup is built around a single sample table to allow switching between modes of operation without moving the sample. Thanks to the large X-ray brilliance from our 3 GeV storage ring, ForMAX will extend conventional studies to the time-resolved realm, thus allowing in situ material processing to be followed.
![[image2]](https://www.iucr.org/__data/assets/image/0014/155120/image2.jpeg)
Focus on dynamics and in situ studies
The superior performance of X-rays from small-emittance storage rings, such as those generated from our 3 GeV accelerator, create opportunities to develop analytical X-ray tools that rely on highly brilliant and coherent X-rays.
These can translate into providing experimental capabilities to tackle many scientific questions ranging from life sciences to nanoelectronics:
(i) For example, protein crystallography can benefit from intense X-ray beams with very small beam sizes at our BioMAX [1] and MicroMAX beamlines, thus providing more measurement opportunities with protein crystals of a small size. Previously challenging membrane proteins are essential for understanding illnesses such as Parkinson's and Alzheimer's disease.
(ii) A larger X-ray brightness opens unprecedented opportunities to perform time-resolved in situ studies. An excellent example is ambient-pressure X-ray photoelectron spectroscopy (AP-XPS) at the HIPPIE beamline [2], where the spectroscopic details of gas molecules reacting on a catalytic surface can be followed under high-pressure conditions. As surface dynamics often change with pressure, it is critical to have access to realistic experimental conditions. A development with Oslo University's users is a novel hardware/software interface for time-resolved AP-XPS experiments. The combination of methods offers complementary spectroscopic and kinetic data about complex reaction mechanisms at surfaces.
(iii) A recent example is an experiment on the deformation dynamics of steel at the DanMAX beamline, which is of great importance for the steel beams used in a car to protect passengers in case of an accident. The speed of loading rate of the steel has an important impact on the resulting mechanical transformations that occur and has not been studied in this realistic way before. The very intense hard X-ray beam penetrates through the sample collecting data from its atomic scale structure while a loading rig simultaneously pulls it.
(iv) Nanoelectronics and energy materials are other areas that can tremendously benefit from focusing incident X-ray probes to small, micrometre and submicrometre sizes. A recent study using our NanoMAX beamline [3] addresses ferroelastic domains of metal halide perovskites, a promising material for future solar cells and light-emitting diodes.
(v) Small-emittance storage rings offer unprecedented opportunities to continue developing tools that exploit the large degree of X-ray coherence of these highly brilliant beams. This is, for example, the case with X-ray photon correlation spectroscopy (XPCS), which is being implemented at our CoSAXS beamline [4] and can uniquely probe the structural equilibrium and out-of-equilibrium dynamics of disordered amorphous matter, such as that found in soft-matter systems.
More examples can be found in the last edition of our annual science Highlights.
An advanced accelerator complex with a 3 GeV MultiBend Achromat lattice storage ring
The MAX IV linear particle accelerator (linac) injects full energy into two storage rings of 1.5 and 3 GeV. They are reliable sources of X-rays with remarkable uptime of about 97% and each delivering more than 4000 beamline hours per year. This unique accelerator complex covers the production of X-ray photons over a broad energy range from vacuum ultraviolet (VUV) to hard X-ray (4 eV to over 40 keV) with no gaps in the photon energy spectrum. It is also possible to compress the electron bunches and feed a set of undulators at its end, producing X-ray pulses as short as 100 fs with a 10 Hz repetition rate to the FemtoMAX beamline [5]. The linac can also be used to seed a future soft X-ray free-electron laser.
![[image3]](https://www.iucr.org/__data/assets/image/0015/155121/image3.png)
The 528-metre circumference lattice of our 3 GeV ring has 20 bending sections or achromats, each including seven bending magnets in a MultiBend Achromat (MBA) structure instead of the two that were the standard until now for synchrotron sources of preceding generations. Based on this design, our 3 GeV ring was the first worldwide realization of a fourth-generation light source. Its small emittance laid the foundation for continuing developing tools that rely on X-ray beams with high brightness and a large degree of coherence.
The implementation of this novel lattice design posed several challenges that had to be solved, such as compact groups of magnets being machined into a common iron block and the space between magnet poles only allowing for a very narrow vacuum chamber between them. As an additional example, the inside of the entire vacuum chamber of our 3 GeV ring is covered in a non-evaporable getter coating to reach the necessary ultra-high vacuum conditions (https://cerncourier.com/a/max-iv-partnership-is-the-key/).
Most light sources around the world are either operating [ESRF (France), Sirius (Brazil)] or planning [Soleil (France), SLS (Switzerland), Diamond (UK), ALBA (Spain), APS & ALS (USA), SPring8 & NanoTerasu (Japan)] to use a hybrid-MBA design as inspiration. An exciting future of discoveries with these bright and coherent X-ray beams from fourth-generation light sources is ahead.
Contact for more information:
Aymeric Robert, Science Director for Physical Sciences MAX IV, aymeric.robert@maxiv.se Marjolein Thunnissen, Science Director for Life Sciences MAX IV, marjolein.thunnissen@maxiv.seReferences
[1] Ursby, T., Åhnberg, K., Appio, R., Aurelius, O., Barczyk, A., Bartalesi, A., Bjelčić, M., Bolmsten, F., Cerenius, Y., Doak, R. B., Eguiraun, M., Eriksson, T., Friel, R. J., Gorgisyan, I., Gross, A., Haghighat, V., Hennies, F., Jagudin, E., Norsk Jensen, B., Jeppsson, T., Kloos, M., Lidon-Simon, J., de Lima, G. M. A., Lizatovic, R., Lundin, M., Milan-Otero, A., Milas, M., Nan, J., Nardella, A., Rosborg, A., Shilova, A., Shoeman, R. L., Siewert, F., Sondhauss, P., Talibov, V. O., Tarawneh, H., Thånell, J., Thunnissen, M., Unge, J., Ward, C., Gonzalez, A. & Mueller, U. (2020). J. Synchrotron Rad. 27, 1415–1429.Copyright © - All Rights Reserved - International Union of Crystallography