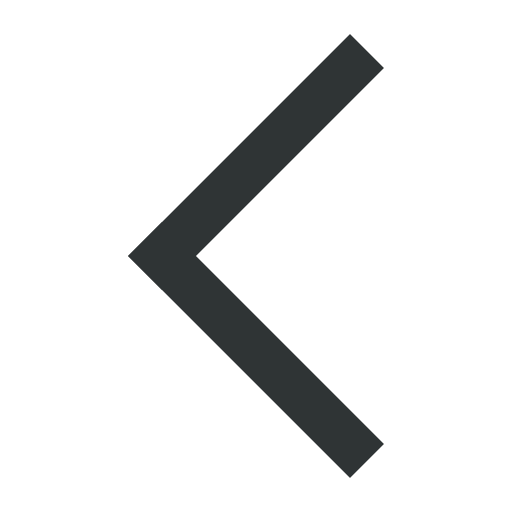
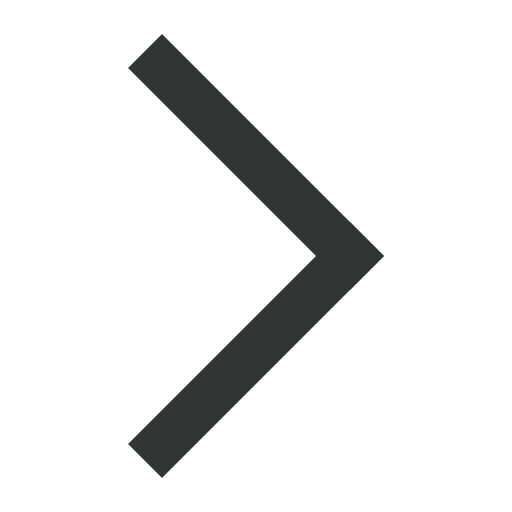


Special report
Overview of crystallography in Japan (1913 – 1980)
Modern crystallography in Japan started right after Laue’s discovery of diffraction of X-rays by crystals (1912), and many valuable achievements were realized during those early years. To our regret, most of them were not recognized outside of Japan. This article will present an outline of crystallography in Japan, especially crystal structure determination, from its advent to approximately 1980. The reviewer apologizes that he cannot fully review research in physics and mineralogy since he is a chemist.
The early years of Crystallography to about 1945
![[Nishikawa]](https://www.iucr.org/__data/assets/image/0015/6702/Image3.gif)
![[Asbestos fiber]](https://www.iucr.org/__data/assets/image/0017/6704/Image5.gif)
![[Nitta]](https://www.iucr.org/__data/assets/image/0016/6703/Image2.gif)
![[Ito]](https://www.iucr.org/__data/assets/image/0018/6705/Image4.gif)
In 1924 T. Ito, who studied crystallography with P. Niggli and later with W. L. Bragg, organized a research group on mineralogical crystallography at the Univ. of Tokyo. In 1933, Nitta organized a crystallographic research group at Osaka Univ.
(1945 – 1980)
Diffraction physics : N. Kato experimentally and theoretically studied X-ray dynamical diffraction, section topographs and secondary extinction. He was President of IUCr (1978~1981), and a winner of the IUCr Ewald Prize. K. Kohra was the principal crystallographer working on the construction of the Tsukuba Photon Factory, which is still one of the most productive SR sources in the world.
Mineralogical crystallography: from 1945 – 1955 T. Ito and his collaborators, R. Sadanaga, Y. Takeuchi, N. Morimoto and others, determined crystal structures of important minerals. Ito developed a powerful indexing method for X-ray powder diffraction patterns, the Ito method. During this period, Ito established the general idea of ‘cell twin’ or ‘Ito twin’ for ‘repeated twinning of unit cell level’. This twin was quite different from the macroscopic one. These results were published in X-ray Studies on Polymorphism (Maruzen, 1950).
Sadanaga and others systematized Ito’s theory of the twin space group. Takeuchi studied the crystal structures of minerals under high pressure and/or high temperature. Morimoto, M. Tokonami and Y. Koto studied structures, superstructures and phase-transitions of many minerals.
Instruments: One of the first large computers built in Japan, comparable to IBM systems, that had enough capacity for computing in crystal structure determination came into operation in 1967. A program system UNICS (universal crystallographic computing system) consisting of fundamental programs for structure determination was written by T. Sakurai, T. Ashida and others (1967), and used by many crystallographers. The first Japanese computer-controlled four-circle diffractometer was produced in 1968 by Rigaku. Then the precision as well as the speed of X-ray single crystal structure determination in Japan improved dramatically. Tsukuba photon factory 2.5GeV came into operation in 1983. It provided a large step forward for the development of crystallography in Japan which saw an ensuing growth in the number of researchers involved in structure determination.
Anomalous dispersion: Using anomalous dispersion Y. Saito and collaborators (1954) were among the first to determine absolute configuration with their work on the complex ion [Co(en)3]3+. Y. Okaya, Saito and R. Pepinsky proposed the theoretically attractive Ps(u) function. Okaya and Pepinsky formulated the relationship between structure factor phases and anomalous dispersion terms. This relationship is used in the phasing method which is now most widely applied for protein crystallography.
Electron density distribution: Y. Tomiie et al. (1958) studied precise electron density distribution in diformylhydrazine and obtained a reasonable coincidence with the one calculated by the MO method. He also found the electron density of a lone pair of electrons belonging to the O atom. Saito, F. Marumo and others studied precise 3d electron distribution in some transition metal ions and compared their results with theoretical predictions. Saito, H. Iwasaki and others studied electron density distributions in charge transfer complexes.
Important compounds: Crystal structures of many important compounds, such as natural organic compounds, coordination compounds, organometallics (A. Shimada, N. Kasai and N. Yasuoka), charge transfer complexes, synthetic polymers (H. Tadokoro), oligopeptides (Ashida), etc, have been studied. Many crystallographers are engaged in research in this category, but only a few of them could be presented here for want of space.
Crystalline phase reactions : In 1977, Y. Ohashi and Y. Sasada observed that racemization occurred when crystals of an (S-α-methylbenzylamine)cobaloxime complex were exposed to X-rays. This reaction could be traced by observing changes in electron density with exposure time. Similar reactions were found in the crystals of related compounds and were extensively studied. This research exploited a new attractive research field in chemical crystallography.
Protein crystals: M. Kakudo, Ashida, N. Tanaka and others began protein crystallography in 1965, and reported a 2.3 Å resolution structure of bonito ferrocytochrome c at the IUCr meeting in 1972. Most of the MIR programs necessary were prepared by Ashida. This was the first high-resolution analysis of a protein structure in Japan. In an international collaboration involving the staff of Dorothy Hodgkin’s laboratory in England, N. Sakabe and others studied 2Zn-insulin at 3.1 Å resolution in 1972 and 1.1 Å resolution in 1984. Y. Iitaka and Y. Mitsui and others studied 2.6 Å resolution subtilisin inhibitor in 1979. Katsube’s group reported the structure of ferredoxin in 1981. From that point on protein crystallography in Japan developed rapidly in both quality and quantity.
Other biological materials: DNA, RNA and related compounds were studied by Y. Mitsui and Iitaka, Sasada’s group and K. Tomita’s group. Bacterial cilia and flagella, muscle, biomembranes, etc have been studied. Among many biophysicists who engaged in the research of these material were T. Mitsui’s group including T. Ueki, and K. Wakabayashi.
Crystallographic community
![[Fujii, Iwasaki, Kato, Tanaka, Ohashi]](https://www.iucr.org/__data/assets/image/0019/6706/Image6.gif)
The Japanese crystallographic community has hosted several successful international meetings including the IXth Congress and General Assembly of the IUCr, Kyoto (1972), the 7th Sagamore Conference, Nikko (1982) and the International Summer School on Crystallographic Computing, Kyoto (1983)
CrSJ and NCC-Japan jointly celebrated the 50th anniversary of crystallography in Japan in Tokyo on July 1, 2000.
(ashida-t@mpd.biglobe.ne.jp)
Activities in big facilities
SPring-8
SPring-8, the largest third-generation synchrotron radiation facility in the world, provides the most powerful synchrotron radiation currently available. The Japanese Atomic Energy Research Institute (JAERI) and RIKEN (The Institute of Physical and Chemical Research) began constructing SPring-8 (Super Photon ring-8GeV) in 1991 with support from Hyogo Prefecture, universities, research institutes and industries, and opened the facility in October, 1997.
Since its completion, all aspects of its operation have been conducted by the Japan Synchrotron Radiation Research Institute (JASRI), which was designated as the sole institute for the management, operation and development of SPring-8. SPring-8 consists of a 1GeV linear accelerator and 8GeV booster synchrotron that generates an incident electron beam of 8GeV and a storage ring (electron energy, 8GeV; current, 100mA; circumference, 1436m) that holds the generated electrons. Figure 3-1-1 shows the Bird’s-eye view of SPring-8.
There are two types of light sources in SPring-8, insertion devices and bending magnets. Insertion devices are either undulators or wigglers. SPring-8 beam ports include insertion device beamlines with a 6m straight section (max. 34, existing 26), long insertion device beamlines with a 30m long straight section (max. 4, existing 1) and bending magnet beamlines (max. 24, existing 21). In-vacuum type undulators developed at SPring-8 have magnet arrays sealed in a vacuum chamber. The arrangement results in a smaller gap between arrays, allowing radiation with shorter wavelength and higher power to be generated.
There is an in-vacuum revolver variable polarization undulator, an in-vacuum figure-8 undulator, a twin helical undulator, a tandem vertical undulator, an elliptical wiggler, and others that generate a variety of polarized radiation. The characteristic photon energy of a bending magnet is 28.9 keV.
62 beamlines are available. 46 are in operation and 2 are under construction. The beamlines are classified as public beamlines, contract beamlines, JAERI/RIKEN beamlines, R&D beamlines, and beamlines for accelerator diagnosis.
Two beamlines, whose lengths are 225m, are already operational at the biomedical imaging center. Two beamlines, longer than 100m, have been developed at the RI laboratory for research on radioactive isotopes and actinide materials. A 1 km-long beamline is used for research on advanced coherent X-ray optics.
The top-up operation was started successfully at SPring-8 in May, 2004. The electron beam is injected at short intervals during user beamtime, so that the current stored in the storage ring is kept constant. The top-up operation has a number of innovative features such as (1) a perturbation-free beam injection scheme, (2) minimum injection beam loss, and (3) a high purity singlebunch beam. This “ideal top-up operation” is used by many other light source facilities around the world. The top-up operation offers the following benefits to users: (1) time averaged brilliance is increased by a factor of two, (2) improvement of thermal stability of the X-ray optics, and (3) a new filling pattern with a high bunch current that was not available in more conventional operations due to the extremely short beam life-time.
SPring-8 invites proprietary and non-proprietary research proposals in June and November and does not charge users for beam time if their research is non-proprietary. The annual operation time of Spring-8 is around 5400 hours, with more than 4200 hours available for experiments. More than 1000 research subjects are accepted annually by SPring-8, and the number of researchers involved exceeds 10000.
Photon factory
![[Q-magnet arrangement]](https://www.iucr.org/__data/assets/image/0003/6708/Image8.gif)
There are 32 X-ray experimental stations out of 58 on the 2.5 GeV ring (energy ranging from 2.2 to 100 keV) and 8 X-ray experimental stations out of 9 on the 6.5 GeV rings (energy ranging from 5 to160 keV). Among those 40 X-ray stations, 22 are for non-biological diffraction/scattering experiments, 5 for protein crystal structure analysis, 2 for small angle scattering, 8 for X-ray spectroscopy, 2 for X-ray imaging and 2 for others. There are 650-700 active experimental proposals every year and approximately 2,700 users repeatedly visit the Photon Factory to carry out their experiments. Eighty to eighty-five percent of proposals are for X-ray experiments, the others are for VUV/soft X-ray experiments. Since the inauguration of the facility in 1982, a number of contributions have been made at the Photon Factory. Those are, for example, MAD analysis of protein crystal structures using synchrotron radiation data, extensive use of image plates for X-ray diffraction experiments, development and extensive use of multi-anvil cells for structure studies under high pressure and high temperature, development of an X-ray Fresnel lens, experimental studies of resonant magnetic X-ray scattering, development of a quarter wave X-ray phase plate using a Bragg-transmitted beam, use of anomalous scattering methods for the study of orbital order in crystals, the development of an in-vacuum undulator and its extensive use for the study of nuclear Bragg scattering, and extensive use of a high energy X-ray elliptical wiggler for magnetic Compton scattering.
To meet requests for more experimental opportunities on insertion device beamlines, the lattice of the 2.5 GeV ring will be modified in the summer of 2005 to increase the number of straight sections for insertion devices. The modifications place new quadrupole magnets of shorter length with higher field gradients closer to neighboring bending magnets to lengthen the existing straight sections or to create new straight sections as shown in Fig. 3-2-1. Four short straight sections of 1.5 m will be created. Furthermore, two 5 m long straight sections will lengthened to 9.2 m, and 8 other sections of 3.5 m will become more than 5 m. Except for one straight section used forelectron beam injection, a total of 13 straight sections will be available for insertion devices on the 2.5 GeV ring. Such an improvement will enable us to install longer undulators with planar or helical magnet configurations and minipole undulators with a narrower gap at the short straight sections. By adding 5 straight sections on the 6.5 GeV ring, the Photon Factory will have a total of 18 straight sections for insertion devices. An in-vacuum short period undulator beamline for structural biology is now being constructed and will be commissioned in the fall of 2005. Another in-vacuum undulator Xray beamline for materials science will be constructed and commissioned in 2006.
Tadashi Matsushita
(tadashi.matsushita@kek.jp)
Neutron facilities
Japan started atomic energy related activities well behind Western countries after World War II. In the mid 1950s the first nuclear research reactor JRR-1 (Japan Research Reactor No.1, 50kW, now preserved in a museum in Tokai) built by the Japan Atomic Energy Research Institute (JAERI) was used for neutron beam research. Neutron scattering research requiring an MW-class reactor started in the early 1960s when JAERI built the JRR-2, which could produce a thermal power of 10MW, thermal neutron flux or 2x1014 neutrons/cm2/sec. There were 9 neutron scattering instruments during its most active period. It was the first neutron source available for inelastic experiments and also played a central role in training many researchers. It was permanently shut down in 1996, as scheduled, after about 35-years of service. Side-by-side with JRR-2, the JRR-3 (10MW, 1x1014 n/cm2/sec, 6 instruments) was built with purely domestic technology for the first time. In the mid 1960s Kyoto University built its 5MW reactor named KUR (3x1013n.cm2/sec, 8 instruments; www-j.rri.kyoto-u.ac.jp/) in Kumatori. It is the only MW-class reactor owned by a university. In late 1960s and 1970s, therefore, three medium-size reactors were available for neutron scattering and other beam experiments while several high flux reactors became operational abroad such as the HFBR at Brookhaven National Laboratory (60MW, 8x1014, USA), HFIR at Oak Ridge National Laboratory (85MW, 1x1015, USA), and HFR at the Institute Laue Langevin (58MW, 3x1015, France). Japanese user’s strong demand for higher neutron flux by the early 1980s culminated in the refurbishment of the JRR-3 to double its thermal power (20MW, 3x1014) and install a new cold neutron source accompanied with a guide hall building. This refurbished reactor renamed as JRR-3M became fully operational in 1990. Currently it supports 25 neutron scattering, 2 neutron radiography and 2 prompt γ-ray analysis instruments and a total number of about 12,000 users per day (www.issp.u-tokyo.ac.jp/labs/neutron/index.html and www.jaeri.go.jp/).
![[Five facilities]](https://www.iucr.org/__data/assets/image/0014/6710/Image10.gif)
![[J-PARC]](https://www.iucr.org/__data/assets/image/0004/6709/Image9.gif)
The Japanese neutron community has been dominated by solid state physicists at the forefront of solid state physics and material science particularly in the field of low-dimensional magnetic and strongly-correlated electron systems including high-Tc superconductors and CMR materials. After cold neutrons became available from JRR- 3M in 1990, however, soft-matter scientists and biologists started using the domestic neutron facilities and now account for as many as one quarter of the total proposals. Another innovation in neutron crystallography is a neutron-sensitive imaging plate (IP) specially developed by the JAERI group with Fuji Film Co., which has opened up a new era of structural biology. Another important instrumental development is the wide variety of neutron optical devices such as the magnetic lens, compound prism, and supermirrors cooperatively developed by RIKEN, JAERI and KUR Groups (http://nop.riken.go.jp/indexJ.html). These devices combined with a new neutron source will lead to new instruments to reach an unexplored region of momentum-energy-spin space for promoting new fields of crystallography.
fujii@neutrons.tokai.jaeri.go.jp
Recent developments in biological crystallography
![[Bovine cytochrome oxidase]](https://www.iucr.org/__data/assets/image/0015/6711/Image13.gif)
![[Sarcoplasmic reticulum calcium pump]](https://www.iucr.org/__data/assets/image/0016/6712/Image11.gif)
![[Bacterial flagellar]](https://www.iucr.org/__data/assets/image/0017/6713/Image12.gif)
![[Sakabe camera]](https://www.iucr.org/__data/assets/image/0018/6714/Image14.gif)
N. Sakabe has been developing IP diffractometers at the Photon Factory since the early 1980s (Fig. 2-2-4). Before SPring-8 began operations, all protein crystallographers were dependent on him for the collection of intensity data. Many Japanese and foreign crystallographers determined a number of novel crystal structures using his detectors at the Photon Factory. The newest version, which has a high quality and high speed detector, was installed at BL-6C in the Photon Factory. S. Wakatsuki has built a new user friendly undulator beamline equipped with a CCD detector. The Photon Factory has one undulator and three bending magnet beamlines and SPring-8 has four undulator and six bending magnet beamlines for protein crystallography. Each beamline has specific features, requiring separate network systems for access. In addition to X-ray beamline facilties, the Japanese Atomic Energy Institute has a neutron beamline facility. A neutron diffractometer dedicated to protein crystals has been developed by Niimura’s group (2004).
Tomitake Tsukihara(tsuki@protein.osaka-u.ac.jp)